Organ-on-a-chip
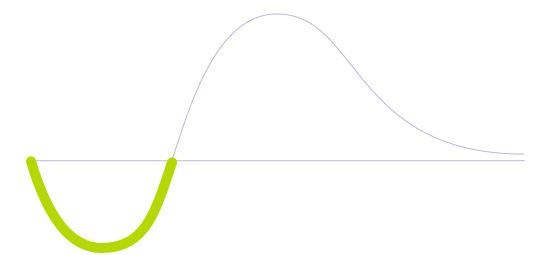
Technology Life Cycle
Initial phase where new technologies are conceptualized and developed. During this stage, technical viability is explored and initial prototypes may be created.
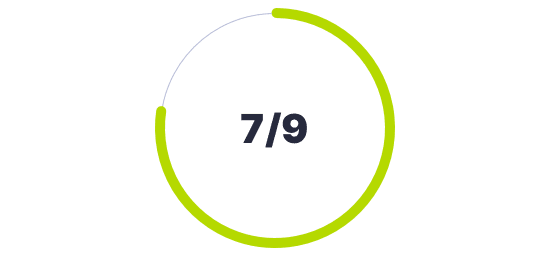
Technology Readiness Level (TRL)
Prototype is fully demonstrated in operational environment.
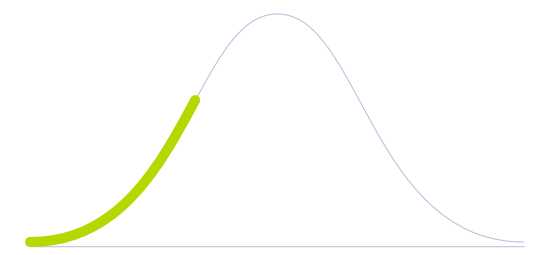
Technology Diffusion
Embrace new technologies soon after Innovators. They often have significant influence within their social circles and help validate the practicality of innovations.
This microfluidic technology involves the fabrication of small 3D devices that mimic the structure and function of human organs. Organ-on-a-chip (OOC) devices consist of a small chip, usually made of plastic or glass, with tiny channels and chambers that can be lined with living human cells, creating a microenvironment that simulates the conditions of the organ it is mimicking.
The technology works by combining microfabrication techniques with tissue engineering to create a functional model of an organ. Cells are seeded into the channels and chambers of the device, where they grow and develop into functional tissue, mimicking the structure and function of the organ in the body.
Organ-on-a-chip technology has the potential to revolutionize the way drugs are developed and tested. It can provide a more accurate and reliable way to test the efficacy and toxicity of new drugs, reducing the need for animal testing and potentially saving millions of dollars in drug development costs. It can also provide researchers with a better understanding of how organs function and how diseases develop, leading to new insights into the underlying mechanisms of disease and potential new treatments.
Moreover, the OOC technology can also be used to study personalized medicine, as researchers can create models that are specific to a patient's genetics and medical history, which can help in the development of personalized treatments.
Future Perspectives
The large-scale adoption of organs-on-a-chip could reduce the time necessary to develop new drugs. It creates more room for experimenting with innovative formulas while abiding by ethical codes throughout the world. Animal and human testing could become something of the past while maintaining industry efficiency.
As the technology matures, these chips could be available as point-of-care testing. That is, at the time and place of patient care, especially in critical care settings such as the intensive care units, the operating room, and the emergency department.
Eventually, such chips could be made on a personalized scale. Every single person could have their own set of chips, with each of them representing a particular organ. Enter personalized tests: drugs and cosmetics could be first tested on the chip to check for incompatibilities and potential reactions and only applied directly to the organism following satisfactory results. Unintended consequences, which create health issues and general frustration with personal care products, could be significantly reduced. Finally, the ultimate goal of organ-on-a-chip technology might be integrating numerous organs into a single chip. From this moment onwards, it would be possible to build a more complex multi-organ chip model, finally achieving a "human-on-a-chip" aspiration.
Image generated by Envisioning using Midjourney