Silicene
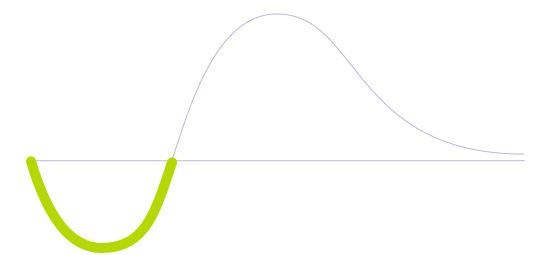
Technology Life Cycle
Initial phase where new technologies are conceptualized and developed. During this stage, technical viability is explored and initial prototypes may be created.
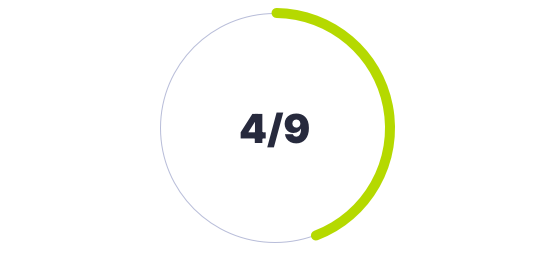
Technology Readiness Level (TRL)
Experimental analyses are no longer required as multiple component pieces are tested and validated altogether in a lab environment.
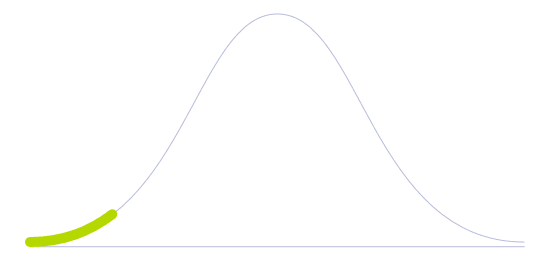
Technology Diffusion
First to adopt new technologies. They are willing to take risks and are crucial to the initial testing and development of new applications.
Carbon and silicon are very similar elements. They lie next to each other on the periodic table — the former is the fundamental structure of organic matter, and the latter is the basis of electronics. Silicene, silicon's two-dimensional allotrope, consists of a hexagonal honeycomb structure, similar to graphene, and can be used for a variety of applications. It is considered a promising material for future electronics because it has unique electronic and optical properties that make it suitable for applications such as field-effect transistors, sensors, and energy storage devices.
One of the main advantages of silicene is its compatibility with silicon-based electronics, which are currently the industry standard. Meaning this material could potentially replace silicon in certain applications, such as transistors, semiconductors, and energy storage devices, because it has higher electron mobility, elevated surface area, and electrical conductivity. Additionally, silicene has potential applications in sensing and detection, as it is highly sensitive to changes in its environment. It also has unique optical properties that make it useful for developing optoelectronic devices.
However, one of the main challenges facing silicene research is its instability, as it tends to react with oxygen and other chemicals in the environment, which can degrade its properties in a matter of seconds. Researchers are working on finding ways to stabilize silicene and improve its properties, such as by using protective layers or modifying its structure.
Future Perspectives
Many silicene applications have yet to be discovered. Its ability to perform logical operations – a significant advantage over graphene – makes it much more suitable for electronics. It could indeed provide a reliable way to integrate semiconductors and transistors in considerably smaller (and faster) components than what we have today. It could be integrated with wearable technologies – applied to the human body –or in sensors, providing ubiquitous nano-sized intelligence.
Image generated by Envisioning using Midjourney