Superhemophobic Material
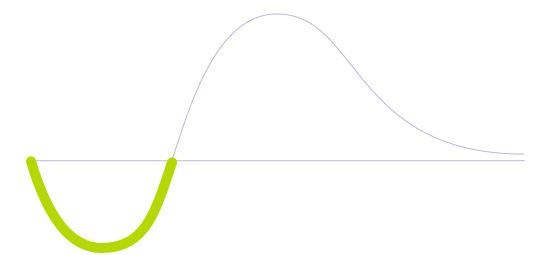
Technology Life Cycle
Initial phase where new technologies are conceptualized and developed. During this stage, technical viability is explored and initial prototypes may be created.
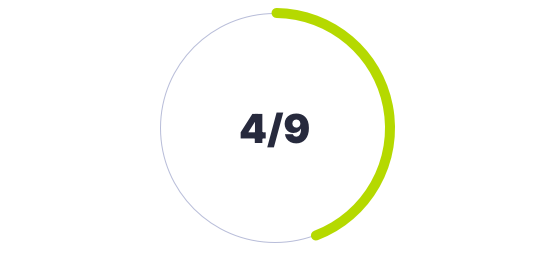
Technology Readiness Level (TRL)
Experimental analyses are no longer required as multiple component pieces are tested and validated altogether in a lab environment.
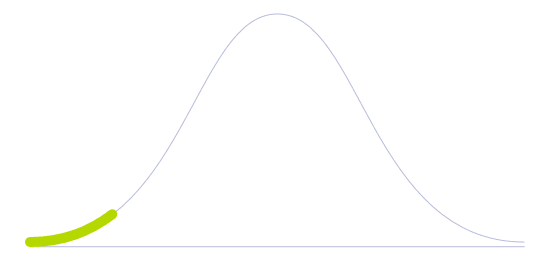
Technology Diffusion
First to adopt new technologies. They are willing to take risks and are crucial to the initial testing and development of new applications.
A titanium-based material that can repel virtually any liquid, but in particular blood, by roughening its surface with nanotubes. By using a substrate made out of superhemophobic titania nanotubes, scientists can use a stable substance that could considerably decrease the surface absorption of protein while simultaneously delaying the clotting process.
This is a key innovation for medicine in terms of implants and medical devices as it can help solve issues such as blood clotting, as blood is incredibly difficult to repel from other materials and surfaces, creating significant challenges for scientists. Some reasons include the high propensity of blood to activate intrinsic hemostatic mechanisms, as well as the induction of coagulation and the activation of platelets upon contact with foreign bodies. In other words, when coagulation is imbalanced, patients using implants or medical devices could suffer from thrombogenesis or the formation of blood clots. In turn, this can impede the blood flow either at the site or even further downstream, causing emboli or exposing the tissue to ischemia and infarction.
The research regarding the interactions of superhemophobic surfaces and blood showed a considerable amount of protein adsorption and impressive platelet adhesion, which indicates this is a potential method for enhancing the compatibility of blood with other materials. The concept of strengthening hemocompatibility has been paramount in scientific research. This is because currently proposed methods, including modified polymer surfaces, did not show the same results as titania nanotubes arrays. Superhemophobic surfaces are a novel approach designed to enhance hemocompatibility; however, the interactions of blood components with these surfaces have not been studied in-depth, and further research is required for proper deployment.
In addition to medical devices, scientists are also considering the development of superhemophobic textiles, but using another substrate besides titanium nanotubes. Research has shown that a chitosan-aloe vera bio-nanocomposite could be used to produce not only blood-repellent but also antimicrobial lab garments, an achievement that could improve the life and treatment of hospital patients in the event of contamination.
Future Perspectives
With the use of blood-repellent materials such as the one based on titania nanotubes, patients can reduce side effects and be protected from contact between foreign devices and blood. However, more long-term research is necessary to fully deploy implantables benefitting from this technology. Another challenge faced by scientists is the development of manufacturing processes suitable for implantables and finding ways to make such devices scalable to attend to all patients with such demands.
Image generated by Envisioning using Midjourney